Ammonia: Fuel vs. Hydrogen Carrier
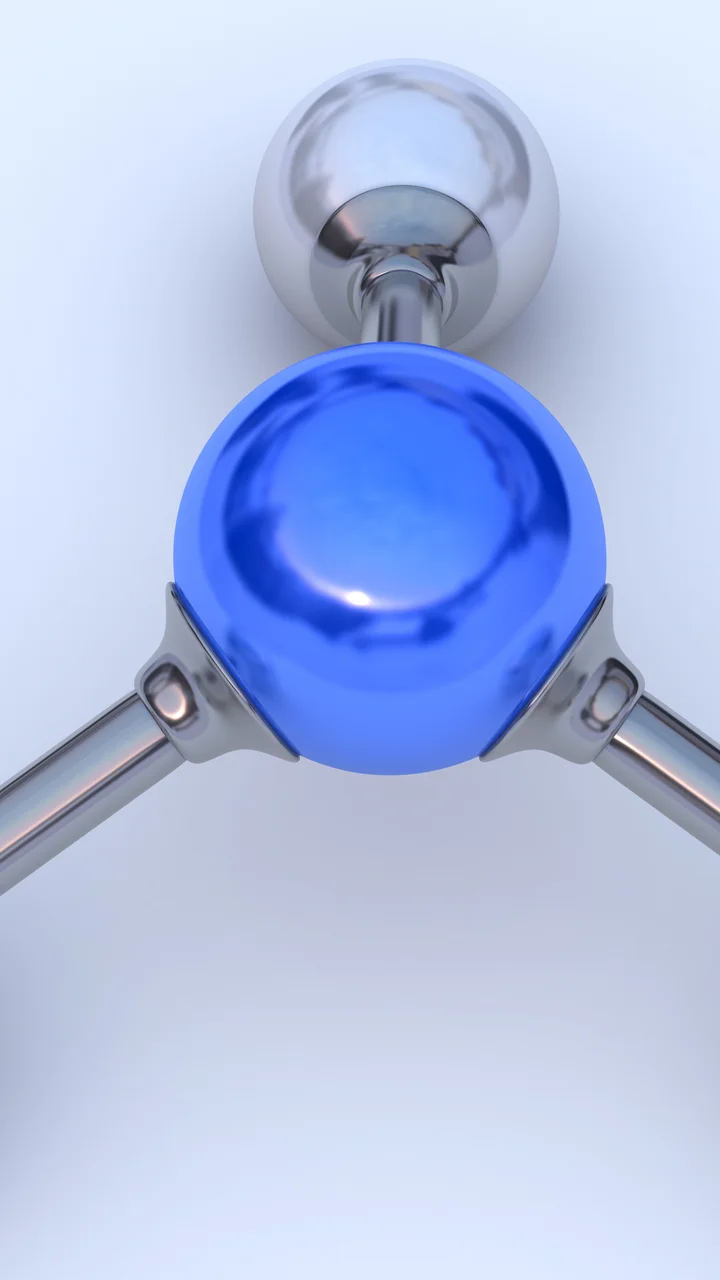
Introduction
The drive to reduce carbon footprint has been taken more seriously over the last few years. More specifically, many global companies have been announcing their strategies to achieve low-carbon or net zero strategies, which will require the use of alternative fuels. Two fuels contending for the lead alternative fuel are hydrogen and ammonia.
While hydrogen has the advantage of high energy density (on a mass basis), the large storage volumes required, and limited existing infrastructure are considered stumbling blocks in the hydrogen as fuel economy. One solution to this hurdle is to consider a hydrogen carrier, such as ammonia, for the transportation and storage of the fuel and crack it back to hydrogen at the user.
The use of ammonia as a hydrogen carrier creates a new question. What is the better fuel – ammonia as a stand-alone fuel or hydrogen from cracked ammonia? At the moment, there is not a clear-cut answer to the question. Rather, the best fuel choice is a function of intended use, quantity, and technology maturity of the fuel user.
Ammonia as Fuel
History of Ammonia as Fuel
Using ammonia as fuel for internal combustion (IC) engines dates back to the early 1800s and saw a surge in use as an alternative fuel during World War II, when oil stockpiles became low. Despite this early start, limited research on IC and spark ignition engines using ammonia as fuel had been conducted until the 2010s. [1]
Utilization of ammonia for marine fuel has been more aggressively researched since 2007. In fact, fuel system developers such as Alfa Laval and engine developers such as Japan Engine Corporation have announced plans to include ammonia in their offerings. [2] Furthermore, MAN Energy has announced efforts to produce ammonia driven marine operations. [3]
Until recently, use of ammonia in fuel cells has been limited due to the tendency of ammonia to dissolve the cell membrane. Advances in solid-oxide fuel cells (SOFC) and polymer electrolyte membrane fuel cells (PEMFC) are proving ammonia as a fuel for fuel cells can be a viable option.
Technology Challenges
Some modifications to IC engines are required for operation on ammonia, which is the first technical challenge for this fuel. More specifically, the space required for fuel storage is typically larger and some material selection modifications are required. Furthermore, higher compression ratios are required, often resulting in a dual fuel injection (e.g. ammonia + diesel, ammonia + hydrogen) to keep the compression ratio modest. In addition, dual fuel applications have been considered to improve flame stability and balance CO and NOx emissions.
As alluded to above, the second challenge faced by ammonia as fuel is NOx emissions. A clear understanding of an engine’s mode of operation is highly important to mitigating NOx and unburnt ammonia in the exhaust gas. For example, some studies have shown NOx emissions exceeding 1000 ppm for engine operation on ammonia. Research has progressed to show that these high NOx emissions can be significantly reduced with engine design and systematic tuning. More specifically, studies have shown the importance of combustor inlet temperature, injection port location, fuel blend, and air injection rate on NOx and ammonia emissions [1],[2],[3],[4],[5]. Proper tuning of engine operation will be highly important as this technology progresses in order to appropriately size downstream selective catalyst reduction (SCR) units needed to remove NOx from the flue gas. Additional details regarding this topic will be discussed in a subsequent paper.
Hydrogen as Fuel
Hydrogen has been demonstrated as a fuel for centuries. In fact, the first hydrogen fueled IC engine was built in the early 1800s. It wasn’t until the early 1900s that an interest in hydrogen fueled engines resurfaced, among other uses such as lift gas for airships. Most recently, hydrogen is commonly used for rocket fuel and for demonstration of fuel-cell vehicles. While turbines run on hydrogen have been demonstrated, they typically require a fuel blend. The density of hydrogen requires much higher flame speeds than hydrocarbons for proper flame propagation in the combustion zone. Consequently, combustors have to be specifically configured for hydrogen as the fuel. [9] The more efficient use for hydrogen in vehicles is with fuel cells. To date, hydrogen fuel cell powered cars are commercially available from three vehicle brands.
Technology Challenges
The production of hydrogen from cracked ammonia is straight forward, and commonly done for industrial applications, such as metal nitriding applications, using heterogeneous catalysis. Ammonia is passed through a heater and then passed over a catalyst bed to use oxidation of ammonia (ammonia decomposition into nitrogen and water) to supplement the endothermic decomposition of ammonia into hydrogen and nitrogen. Existing cracker technology is readily available in the 1-2 ton/day range[10] from companies such as Lindberg/MPH, CI Hayes, Koyo Thermo, and Sergeant & Wilbur, Inc. Small scale cracking applications have been proposed but are not commercially available [11]. The challenges faced for cracking technology advancements is twofold. First, existing crackers use a nickel-based catalyst which is not efficient and requires high temperatures. Current research is focused on ruthenium, cobalt, and lithium catalysts that can operate at lower temperatures. Secondly, as the crackers become larger, developers are faced with the challenge of achieving the proper heat transfer to the catalyst.4
Another ammonia decomposition method that is still in the research phase is plasma decomposition. Similarly, hydrogen can also be produced from ammonia using ammonia electrolysis. In this mechanism, an alkaline electrolytic cell is used to couple ammonia elecro-oxidation and hydrogen evolution. To date, the process has been determined to be too slow for practical implementation.
Energy and Capex Comparison
The mass energy density of hydrogen is 120 MJ/kg as compared to 18.6 MJ/kg for ammonia, hence its popularity as an alternative fuel. However, once the energy losses due to heating, cracking, and post polishing (i.e. removal of residual ammonia) is considered, the available energy of the hydrogen from cracked ammonia is nearly the same as that of original ammonia. Take for example, 1 ton of ammonia. If utilized as fuel, 2% of the ammonia would be used for the operation of the SCR and the remaining 98% would produce 16,530 MJ of energy. This same 1 ton of ammonia would produce 0.18 tons of hydrogen which is equivalent to 19,205 MJ of energy. However, approximately 15% of this energy is required for the cracking/processing operation for a net energy production of 16,626 MJ.
If the energy output is nearly the same, what about capital expenditure? For this exercise the cost of an SCR unit was compared to the cost of a cracking unit for a particular volume of ammonia per day.
The SCR costs used for this analysis were extrapolated from a variety of sources for power plant installations [12],[13]. In addition, the impact of initial flue gas NOx concentration on cost was estimated using the EPA Air Pollution Control Cost spreadsheet[14]. The cost of an ammonia cracking unit is more uncertain, especially at large sizes that aren’t currently commercially available. Cracker costs metrics were obtained from other alternative fuel studies, primarily completed for the marine industry and a budgetary quote for a small unit3,7,[15]. Cost were calculated for an SCR and Cracking Unit for ammonia volumes up to 1000 tpd. If engine development for operation on ammonia progresses to where outlet NOx is nearly the same as current operation on natural gas, SCR installation was calculated as the cheaper option over the entire range. However, with some studies documenting as high as 1000 ppm NOx for turbine operation on ammonia, the calculated cost for an SCR unit to remove these higher levels indicates ammonia cracking for larger ammonia quantities (around 200 tpd and larger) is the more economical choice.
It is important to note that this cost calculation did not consider the cost of ammonia production, only the cost associated with an SCR or an ammonia cracking unit. Furthermore, it did not consider engine technology maturity (i.e. if an engine designed for ammonia or hydrogen operation is currently commercially available), fuel transport and storage availability, required fuel conditions (temperature and pressure), or SCR/Ammonia Cracker technology size availability. Consequently, while the economics suggest ammonia as a fuel may be preferred, the choice between fuel or hydrogen carrier will ultimately be determined by an individual need based on overall system maturity.
Conclusion
In summary, both ammonia and hydrogen are likely to have a place in net zero strategies. Based on a high-level cost comparison, with further technology development the use of ammonia as a fuel rather than a hydrogen carrier should become the more economical choice. However, the available size and maturity of ammonia engines, turbines, or fuel cells as compared to the size and maturity of ammonia crackers, hydrogen engines, and fuel cell will need to be weighed on an individual basis for each intended application.
[1] A review of ammonia as a compression ignition engine fuel. Dimitriou, Pavlos and Javaid, Rahat. International Journal of Hydrogen Energy. 15(2020)7098-7118.
[2] A Preliminary Study on an Alternative Ship Propulsion System Fuel by Ammonia: Environmental and Economic Assessments. Kim, Kyunghwa et al. Journal of Marine Science and Engineering. March 7, 2020.
[3] The Potential Role of Ammonia as Marine Fuel—Based on Energy Systems Modeling and Multi-Criteria Decision Analysis. Hansson, Julie et al. Sustainability, April 17, 2020.
[4] Ammonia for Power. Valera-Medina, A, & et.al. Progress in Energy and Combustion Science (2018), 63-102.
[5] Ammonia, Methane, and Hydrogen for Gas turbines. Valera-Medina, A., et. Al. Energy Procedia (2015), 118-125.
[6] Performance of Ammonia-Natural Gas Co-Fired Gas Turbine for Power Generation. Ito, Shintaro. 2018 AiChE Annual Meeting.
[7] American Energy Conference 2020, Panel 1. Presentation from Starfire Energy.
[8] Science and Technology of Ammonia Combustion. Kobayahi, Hideaki et al. Proceeds of the Combustion Institute 37(2019),109-133.
[9] Power to Gas: Hydrogen for Power Generation. Fuel Flexible Gas Turbines as Enablers for a Low or Reduced Carbon Energy Ecosystem. GEA3861. February 2019.
[10] Ammonia to Green Hydrogen Project: Feasibility Study. Siemens, ENGIE, Ecuity, and Science & Technology Facilities Council, April 2020.
[11] Ammonia as an Alternative Energy Storage Medium for Hydrogen fuel Cells: Scientific and Technical Review for Near-Term Stationary Power Demonstration Projects, Final Report. Lipman, Tim and Shah, Nihar. Research Report UCB-ITS-TSRC-RR-2007-5. November 2007.
[12] Estimating SCR Installation Costs, Power (2006).
[13] Selective Catalytic Reduction, Chapter 2. Sorrels, John. May 2016.
[14] Cost Report and Guidance for Air Pollution Regulations. EPA, url: https://www.epa.gov/economic-and-cost-analysis-air-pollution-regulations/cost-reports-and-guidance-air-pollution
[15] Lindberg/MPH Model 16-RO-150-HYAM; budgetary quote November 2020.
Contact Us
Looking for a partner in innovation?
Let's Talk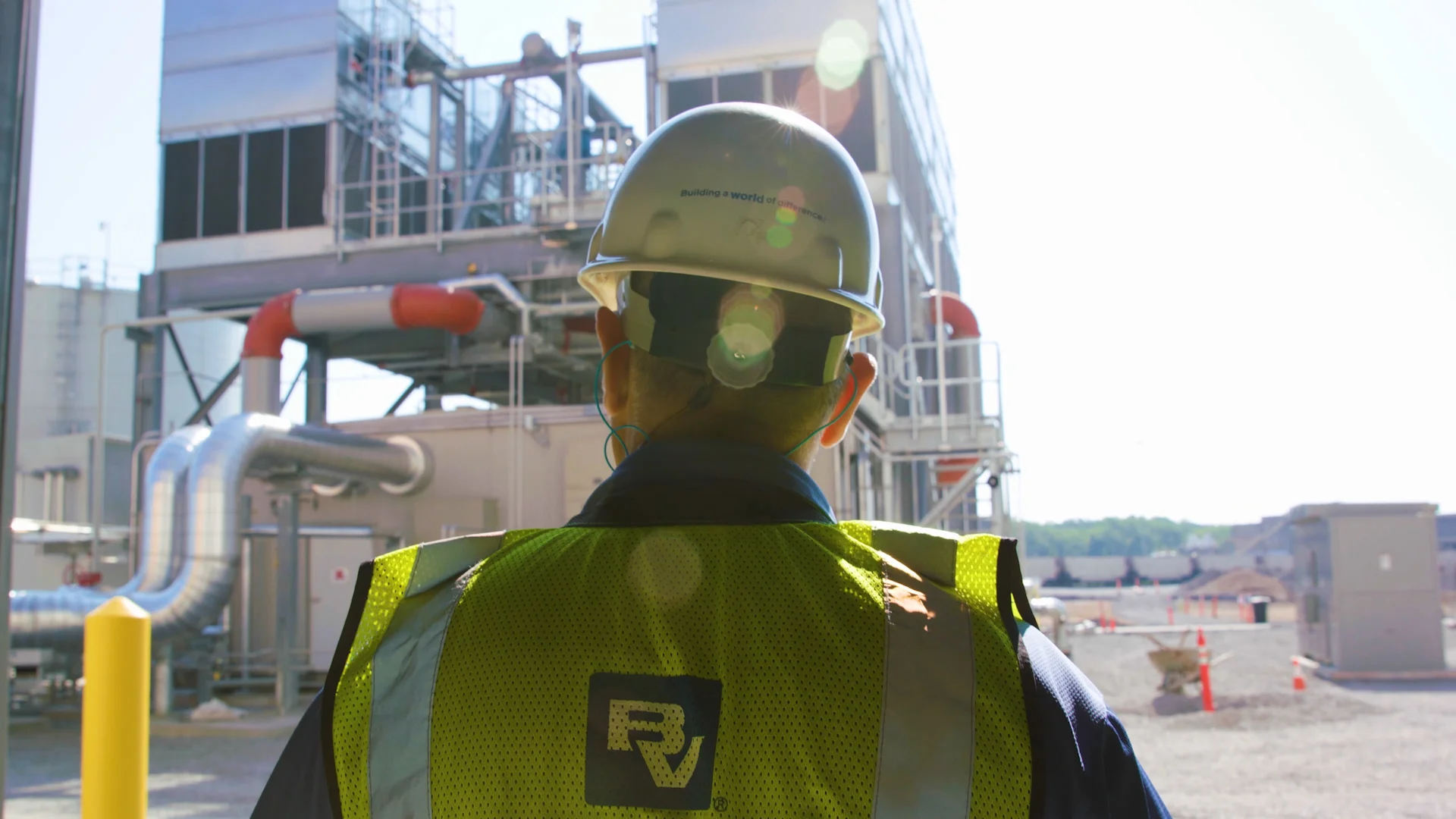